Electroceramics
While ceramics have traditionally been admired for their mechanical and thermal stability, their unique electrical, optical and magnetic properties have become of increasing importance in many key technologies including communications, energy conversion and storage, electronics and automation. Such materials are now classified under Electroceramics as distinguished from other functional ceramics such as advanced structural ceramics.
Historically, developments in the various subclasses of Electroceramics have paralleled the growth of new technologies. Examples include: Ferroelectrics – high dielectric capacitors, non-volatile memories; Ferrites-data and information storage; Solid Electrolytes – energy storage and conversion; Piezoelectrics – sonar; Semiconducting Oxides – environmental monitoring.
The performance of electroceramic materials and devices depends on the complex interplay between processing, chemistry, structure at many levels and device physics and so requires a truly interdisciplinary effort by individuals from many fields. Topical areas cover a wide spectrum with recent active areas including sensors and actuators, electronic packaging, photonics, solid state ionics, defect and grain boundary engineering, magnetic recording, nonvolatile ferroelectric memories, wide band gap semiconductors, high Tc superconductors, integrated dielectrics and nano-technology. Recent advances in these areas are described in the Journal of Electroceramics.
Examples of electroceramic materials and application
Piezoelectric, Pyroelectric, and Ferroelectric Materials
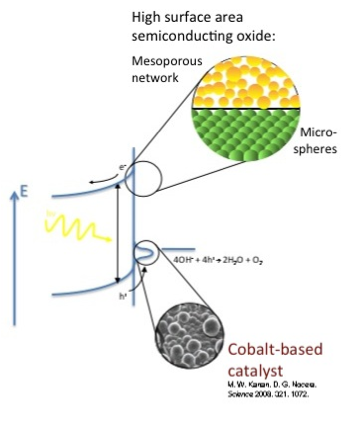
Piezoelectricity is defined as a change in electric polarization with a change in applied stress (direct piezoelectric effect). The converse piezoelectric effect is the change of strain or stress in a material due to an applied electric field. The microscopic origin of the piezoelectric effect is the displacement of ionic charges within a crystal structure. In the absence of external strain, the charge distribution within the crystal is symmetric and the net electric dipole moment is zero. However when an external stress is applied, the charges are displaced and the charge distribution is no longer symetric and a net polarization is created. A material can be only piezoelectric if the crystallographic unit cell has no center of inversion.
In some cases a crystal posses a unique polar axis even in the unstrained condition. This can result in a change of the electric charge due to a uniform change of temperature. This is called the pyroelectric effect.
Ferroelectric materials are a sub-class of pyroelectric materials in which the direction of the electric dipole can be reversed by applying an electric field.

Dielectric materials are insulators used for their exceptional dielectric properties. When a matirial is introduced between two plates of a capacitor, the total charge stored in the capacitor will change. The change depends on the ability of the material to polarize under an electric field. The dielctric constant, or permittivity, of a material determines the change in charge storage. For high capacity applications (‘high-K dielectrics), a high dielectric constant is needed. Since the dielectric constant depends on the polarization in the material, ferroelectric materials are usually the materials of choice.
Electro-optic and Electro-chromic Materials
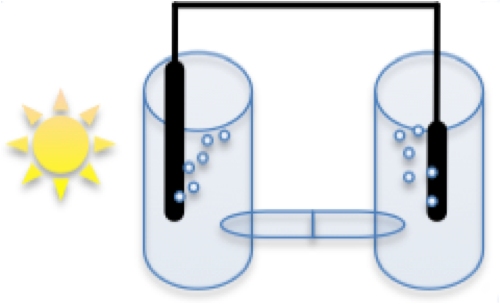
The electro-optic effect is the change in the refractive index as a function of an externally applied electric field. In anisotropic materials, the index of refraction depends on the direction of propagation and the direction of polarization of the light (optical birefingence). This means that the two components of light polarization can propagate at a different speed inside the material. This in turn causes a rotation of the overall polarization direction. Applying an electric field will change the index of refraction by a different amount for the two polarizations, causing further rotation of the polarization vector. By placing the electro-optic material between two polarizers one can control the amount of light passing through by changing the voltage.
Semiconductors, ionic conductors, and mixed electronic/ionic conductors (MIECs)
Most structural ceramics are known for their high resistance to charge transfer, and are therefore useful as electric insulators. Some electroceramics, however, are very useful for the exact oposite reason – they can accomodate charge transfer. Semiconducting ceramics, just like silicon and III-V materials, can be either p-type or n-type. Their electrical conductivity often depends on oxidation-reduction reactions with the surrounding enviroment; these materials are highly useful as chemoresitive gas sensors.
Ionic conduction is achieved by the movement of ions (atoms of positive or negative charge) through the solid. This transfer is usually done via point defects called vacancies in the crystal lattice. Such movement can require high energy, making ionic conduction very strongly dependent on temperature. Ionic conductors are useful in gas sensors, fuel cells, and batteries.
Materials that can accomodate electron conductivity as well as ionic conductivity are known as mixed conductors, and are particularly useful as solid oxide fuel cell (SOFC) electrodes.